Saltwater Pearls from the Pre- to Early Columbian Era: A Gemological and Radiocarbon Dating Study
ABSTRACT
Natural pearls were recovered and treasured by the indigenous peoples of the Americas long before Columbus’s voyages reached the New World. The once-abundant oyster beds of the Caribbean islands off the coast of Venezuela were heavily exploited during Spanish colonization, as pearls were harvested and shipped back to the Old World. A total of 85 saltwater natural pearls reportedly from the pre- to early Columbian era (late fifteenth to early seventeenth century) and found in Central and South America were examined for this study. Gemological and advanced analyses confirmed they were natural saltwater specimens, most likely originating from a Pinctada species. In addition, 14C isotope radiocarbon dating of material from the samples supported the claimed age of these pearls.
INTRODUCTION
Pearls were treasured by the indigenous peoples of the Americas for millennia (Donkin, 1998). These beautiful gems were commonly associated with spiritual power, social rank, and feminine fertility (Saunders, 1999). Pearls were found in the palaces of Montezuma and were prized by the later inhabitants of Mexico and Peru (Kunz and Stevenson, 1908). The discovery of the New World by Christopher Columbus in 1492 and subsequent Spanish colonization radically changed the lives of the natives. Many were enslaved by colonists in pursuit of gems such as pearls, one of the most sought-after commercial products of the period.
The New World’s most productive sources of pearls in the early 1500s were the oyster beds near the Venezuelan islands of Margarita, Cubagua, and Coche, and to a lesser extent in the waters off the Colombian coast and the Gulf of California in Mexico (Mackenzie et al., 2003; Southgate and Lucas, 2011; see figure 1). Within a few decades of the New World’s discovery, the production of natural pearls peaked as a consequence of heavy demand in the Old World. These treasures were shipped to Spain and used in jewelry and ornamentation for the nobility and the Church. During the Renaissance period, pearls and pearl jewelry became extremely popular in Europe due to the influx of significant quantities of saltwater pearls from the newly discovered Americas (Dirlam et al., 1985). Even today, famous historical pearls such as La Peregrina or the Hanoverian pearls owned by Queen Elizabeth I are still in fine condition. Archival records and auction house sales prove that “old pearls” have survived through the centuries. Yet the Spanish failed to understand the importance of conservation and sustainable harvesting (Romero, 2003). Production fell sharply by the mid-1500s due to the scarcity of oysters and as a result of indigenous rebellions (Romero et al., 1999).
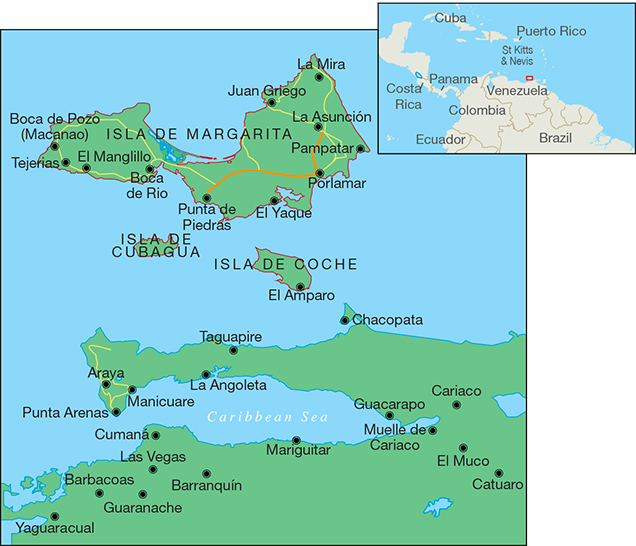
There were attempts to revive pearl harvesting in the 1600s, and a few intermittent fishing activities also took place in the eighteenth and nineteenth centuries, but on a much smaller scale. One can still see the pearling traditions reflected in the coat of arms for Nueva Esparta, the Venezuelan state that comprises the islands of Margarita, Coche, and Cubagua. Shown in figure 2, the emblem depicts a strand of natural pearls. Such pearls are seen in numerous jewelry pieces from this period, as well as in paintings depicting women of all classes wearing multi-strand pearl and coral bracelets, necklaces, and earrings (Pierce and Frick, 2015).
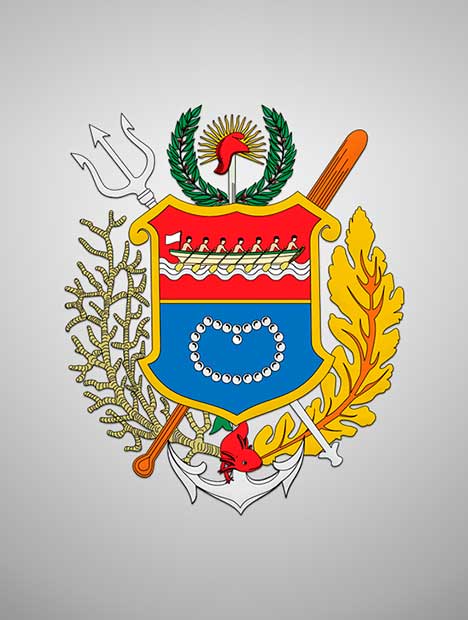
Establishing the ages of such historical pearls requires advanced analytical techniques in addition to routine gemological testing. Radiocarbon (14C) dating is a method for determining the age of an object containing organic material. Three isotopes of carbon occur naturally: 12C and 13C (both stable) and 14C (unstable or radioactive). These isotopes exist in equilibrium and enter into plants and animals through biological food chains until their death. The amount of 14C will then slowly decay with a half-life of 5,568 years (the Libby standard). The remaining concentrations of 14C may be detected using accelerator mass spectrometry (AMS), making it possible to calculate the sample’s age. However, there is a difference between radiocarbon dating of a terrestrial sample and a sample from a marine environment due to the delay in exchange rates between atmospheric CO2 and ocean bicarbonates, and the dilution effect caused by the mixing of surface waters with the very old upwelled deep waters. A global marine reservoir correction using the IntCal Marine13 calibration data set is commonly applied for marine samples. In addition, local marine reservoir corrections (delta R) can be applied if the samples are of known geographic origin. Radiocarbon dating has been widely used in many fields, including history, oceanography, geology, archaeology, and materials science (Taylor et al., 2013), so its importance in determining the approximate age of samples is well established.
The present study focuses on a group of pearls reportedly from the pre- to early Columbian era. They were purportedly collected in Central or South America, most likely off the island of Cubagua, although their history and exact origin are not clear. The pearls appeared to be old, judging from their external form and the worn condition of their nacreous surfaces (figure 3). Gemological examination at GIA and age dating at the University of Arizona and the University of Tokyo confirmed that these were saltwater natural pearls from the pre- to early Columbian era. Meanwhile, the Gübelin Gem Lab conducted an independent study on pearls from the same supplier that were said to be from the same geographic source. Their results are included in this joint study in order to identify the nature and origin of these intriguing gems.
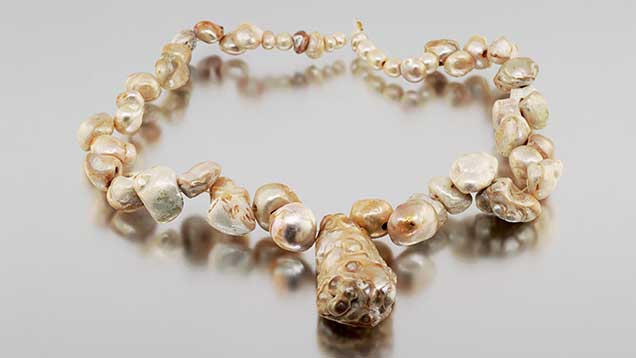
MATERIALS AND METHODS
A total of 75 baroque to semi-baroque pearls ranging from 3.46 × 2.80 × 2.17 mm to 31.41 × 16.86 × 10.25 mm were examined in the study conducted by GIA. According to the supplier, these are part of a large collection of natural specimens reportedly from Central or South America, most likely the waters off Cubagua. It is presumed that they were later found buried underground, although the exact origins were not clear to the supplier. Shell powder taken from one blister pearl in this group was used in 14C radiocarbon dating experiments (figure 4). One small pearl weighing 0.74 ct was also sacrificed for an additional 14C radiocarbon dating experiment.
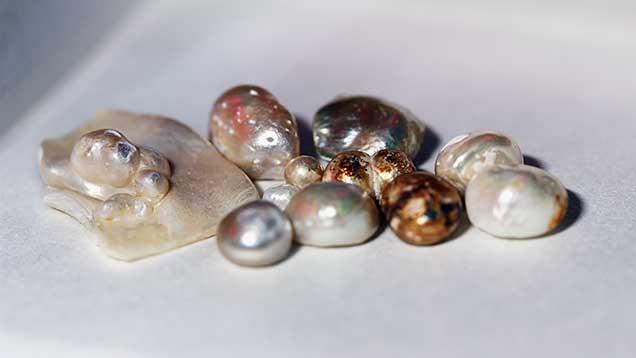
The pearls were examined with a standard gemological microscope, and photomicrographs were obtained using a Nikon SMZ1500 stereomicroscope. Internal structures were examined using a MatriX-FocalSpot Verifier HR FSX-090 2D real-time X-ray microradiography (RTX) unit (45 kV and 0.18 mA excitation). XRF reactions were observed using a MatriX-FocalSpot Verifier PF-100 X-ray fluorescence unit (100 kV and 3.2 mA excitation) equipped with a Canon EOS REBEL T4i DSLR camera (5-second exposure, F5.0, ISO 12800). The chemical composition of 11 loose samples was obtained with a Thermo Scientific ARL Quant’x energy-dispersive X-ray fluorescence (EDXRF) spectrometer. Conditions varied depending on the element of interest: low Za—8 kV voltage, no filter, 50 sec live time; low Zc—12 kV voltage, aluminum filter, 200 sec live time; mid Zc—28 kV voltage, Pd thick filter, 100 sec live time; high Zb—50 KV voltage, Cu thick filter, 50 sec live time.
Radiocarbon dating experiments were carried out at the University of Arizona’s Accelerator Mass Spectrometry Laboratory in Tucson. Shell powder (40 mg) from one blister pearl was ultrasonically cleaned for one hour in several changes of type 1 deionized water (>18 MΩ). Carbon was isolated by acid hydrolysis using 85% phosphoric acid under vacuum. The carbon dioxide was cryogenically purified and quantified manometrically in a known volume. Stable isotope measurements (δ13C) were made offline on an aliquot of sample gas using a VG Isotech dual-inlet stable-isotope mass spectrometer. The carbon stable isotope value was measured against an internal laboratory standard referenced to VPDB, to a precision of ±0.1‰. Graphitization was carried out using Zn and Fe by the method reported by Slota et al. (1987), and AMS measurements were carried out using an NEC 2.5 MeV Pelletron accelerator mass spectrometer. The measurement standards were NIST SRM 4990B and 4990C. The measurements were background-subtracted and corrected for isotope fractionation. The radiocarbon measurements were calibrated to calendar age ranges using the Marine13 database and OxCal 4.2.4 software, with a local marine reservoir correction (delta R). The local marine reservoir correction was estimated by assuming that the true origin of the shell was off the coast of Cubagua Island. A marine reservoir correction database (http://calib.org/calib) was queried, and a local marine reservoir value was calculated as the mean delta R from the five nearest reference samples.
Additional radiocarbon dating experiments were performed at the University of Tokyo’s University Museum on the shell powders from the same blister pearl sample (67.8 mg) and the sawn half of a small natural pearl sample (54.5 mg; see figure 5). Both samples were pretreated with weak hydrochloric acid (0.1 M) to clean their surfaces. After the acid pretreatment, they were pulverized using an agate mortar for carbon purification and graphitization. Each pulverized sample was encapsulated in vacuum vessels with anhydrous phosphoric acid (100%) and reacted at 70°C (Wachter and Hayes, 1985). CO2 gas reaction products were purified using a vacuum line and transferred into the reaction tube containing pure Fe powder. After filling the reaction tube with hydrogen gas (2.2 times an equivalent molar amount of carbon), the reaction tubes were heated to 650°C for six hours (Kitagawa et al., 1993). In order to calculate the conventional 14C age (before present, or BP), the δ13C value for the correction of isotope fractionation was measured simultaneously (Stuiver and Polach, 1977).
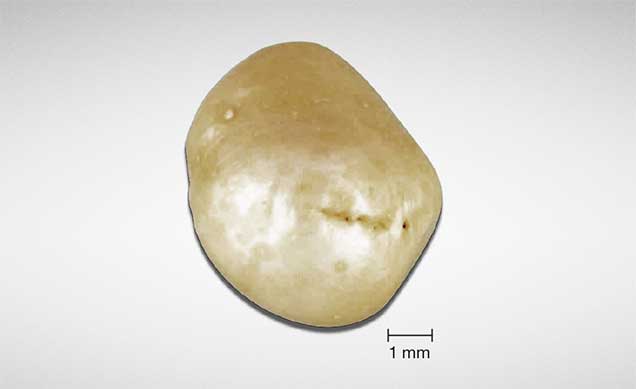
Ten additional pearls, reportedly from the same supplier, were tested independently by the Gübelin Gem Lab in Lucerne, Switzerland. The Gübelin lab used RTX to examine the growth structures and EDXRF chemical analysis to determine the saltwater or freshwater origin. Furthermore, five of these samples were radiocarbon dated at an independent institute in Switzerland.
RESULTS
General Observations. Although the pearl samples were of various shapes and sizes, their external appearances were similar (figures 3–6). Fifty-three of them were drilled and strung as a strand necklace, and the rest were undrilled. The drilled pearls were reportedly drilled before their discovery and only recently strung. The drill hole outlines did appear “handmade,” with primitive jagged edges as opposed to the even and round drill holes created by modern drilling machines. Most of the pearls showed white or off-white (cream) bodycolors, while reddish or brownish stains could be found on the surfaces of a few. Microscopic examination revealed overlapping aragonite platelets typically seen in nacreous pearls, as well as evidence of aging, including delicate chalky outer surfaces, worn and peeled layers, and surface cracks in some cases (figure 7). However, many of the samples still displayed good surface conditions, a high luster, and a strong orient from underlying nacreous layers, reflecting their once-fine quality (figure 8). Additionally, one of the undrilled pearls and the large center pearl in the strand were found to be blister pearls, consisting of multiple small pearls that formed into an aggregate with a cut shell base (again, see figures 3 and 4). These strongly resembled pearls believed to be from the Venezuelan islands that were recovered from a colonial-era Spanish shipwreck off the coast of Florida (Koivula et al., 1992).
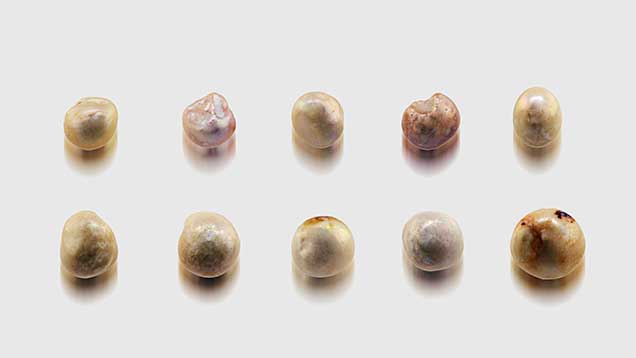
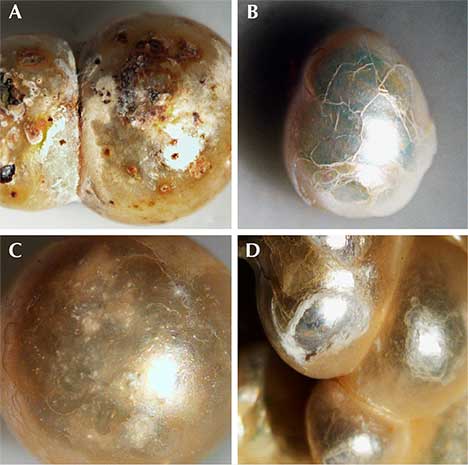
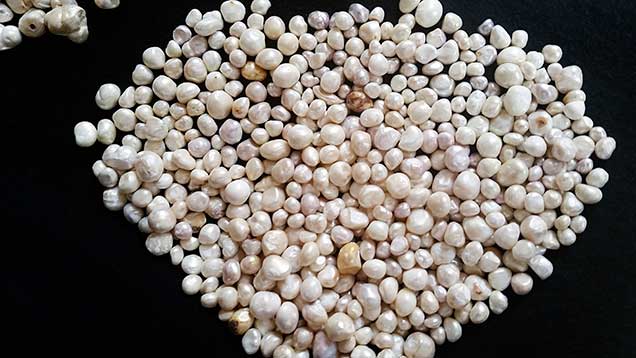
Internal Structures. The pearls in this study exhibited various natural internal structures revealed by RTX, in keeping with many other natural samples tested by GIA. Some had a relatively tight internal structure with only a few faint growth arcs. Twin pearls or pearls with three or more segments were also common in this group, with either natural concentric growth rings or tight structure in each segment separated by boundary or fold features. Other structures observed included natural concentric growth rings and/or dark organic-rich centers with natural growth arcs (figure 9). The central blister pearl in the strand had a very interesting appearance and structure. It was an aggregation of many smaller pearls, and its base was flat, likely the result of being cut from a host shell. Its internal structure correlated with its external appearance, with numerous small growth centers separated by boundary/fold lines. The centers of these small growth units usually contained conchiolin-rich materials that displayed dark areas observed via microradiography (figure 10, left). Similar multiple growth structures were observed in the loose blister pearl from which powder was taken for carbon dating (figure 10, right). On the whole, the internal structures proved these were natural pearls that formed without human interference (CIBJO, 2016).
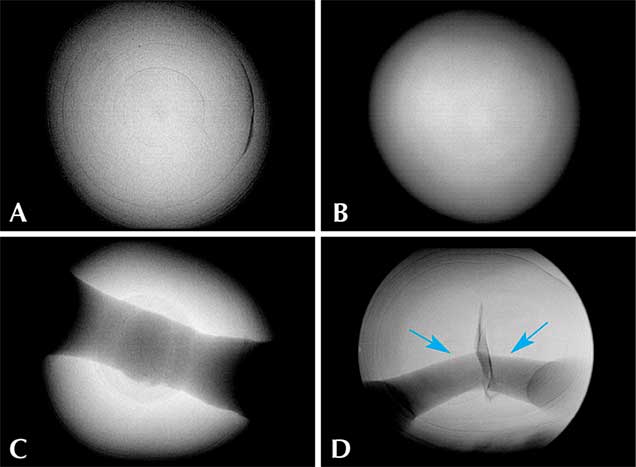
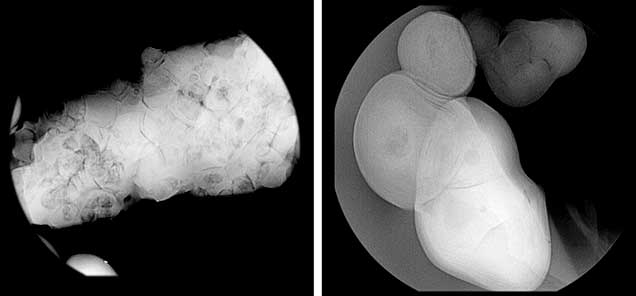
X-ray Luminescence and EDXRF Spectrometry. X-ray luminescence is used to separate saltwater from freshwater pearls owing to the latter’s higher concentrations of manganese (Mn; Hänni et al., 2005, Kessrapong et al., 2017). Freshwater pearls may show obvious greenish yellow fluorescence under X-ray excitation, while saltwater natural pearls are usually inert. Even the freshwater nuclei used in saltwater bead-cultured pearls may be detected, depending on the thickness of their nacre. All the samples tested in this study lacked luminescence under X-ray exposure, indicating that they formed in a saltwater environment. EDXRF qualitative analysis detected only calcium (Ca) as the major element and strontium (Sr) as a trace element in the majority of samples. Very low quantities of Mn were detected, further confirming a saltwater origin. The results are consistent with earlier studies on Mn and Sr concentrations of saltwater pearls from bivalve mollusks (Gutmannsbauer and Hänni, 1994), and the Sr/Mn concentration ratios are generally very high compared to typical freshwater counterparts, similar to previous studies (Karampelas and Kiefert, 2012). However, pearls discolored by reddish and brownish stains were found to possess high levels of iron (Fe) on their surfaces. These stains may have been due to the various storage and environmental conditions the pearls were exposed to throughout their long history, where rust (iron oxide) or other forms of oxidizing contamination found their way onto, and partially into, the surfaces.
14C Radiocarbon Dating. The radiocarbon dating result of the shell powders from a blister pearl sample tested at the University of Arizona is shown in figure 11. The uncalibrated 14C age was measured to be 776 years (+/– 25 years) BP. Using the Marine13 database and OxCal 4.2.4 software with a local marine reservoir correction (delta R), the calibrated calendar age ranges were measured to be between the years 1472 to 1537 (68% probability) and 1455 to 1615 (95% probability).
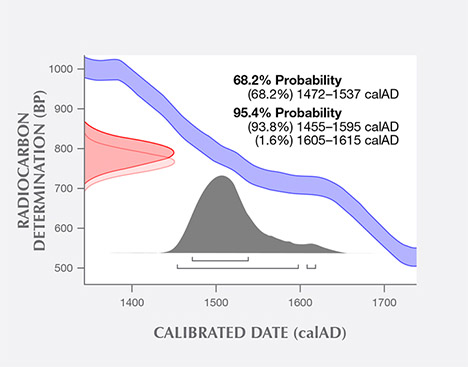
Additional experiments were conducted at the University of Tokyo on a sample of the same shell powder from the blister pearl and one additional pearl sample. The uncalibrated age of the shell powder was 713 years (+/– 16 years) BP, and the uncalibrated age of the pearl sample was measured to be 719 years (+/– 14 years) BP. Using the same database and software as the University of Arizona, the University of Tokyo measured calibrated calendar ages ranging from 1519 to 1616 (68% probability) and 1491 to 1648 (95% probability) for the shell powder (figure 12), and from 1508 to 1615 (68% probability) and 1487 to 1644 (95% probability) for the pearl sample (figure 13). One thing to point out is that due to discontinuous regions of the calibration curve, not all dates in the radiocarbon age range are equally likely. A better probabilistic method is to take the original normal distribution of radiocarbon age ranges and use it to generate a histogram showing the relative probabilities for calendar ages. Local marine reservoir correction for the Caribbean Sea (Wagner et al., 2009) was also included in the University of Tokyo measurements. Whenever possible, it is important to know the geographic location of samples under investigation, as the final data may be affected (from a few years to a few hundred years) by the lack of such valuable information. In summary, the radiocarbon dating results from both laboratories suggested that these samples were from the pre-Columbian or, more likely, early Columbian era, when natural pearl resources in Central and South America were heavily exploited by Spain.
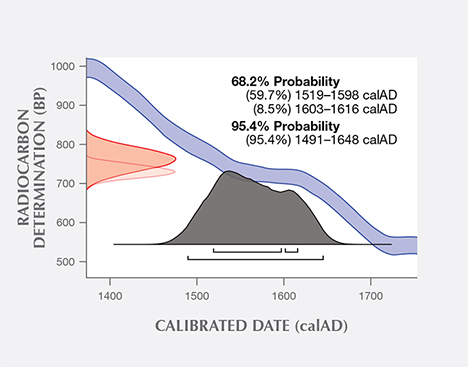

Additional Results Obtained by the Gübelin Gem Lab. Independent testing at the Gübelin Gem Lab of 10 loose pearls, reportedly from the same supplier, supported these results. X-ray microradiography revealed natural growth structures, and EDXRF chemical analysis confirmed the saltwater origin (figure 14). Some of these pearls exhibited a ceramic or claylike residual layer on their surface. This material may have originated from the vessel in which the pearls were stored for several centuries, perhaps a clay jar or clay pot (figure 15). Furthermore, five of the samples were radiocarbon dated at an independent institute in Switzerland, and their results revealed various date ranges (figure 16), with local marine reservoir adjusted for waters off the coast of Venezuela:
- 1504 to 1587 (68% probability) and 1490 to 1635 (95% probability)
- 1468 to 1513 (68%) and 1451 to 1539 (95%)
- 1591 to 1667 (68%) and 1547 to 1677 (95%)
- 1534 to 1618 (68%) and 1508 to 1648 (95%)
- 1543 to 1626 (68%) and 1517 to 1654 (95%)
The results confirmed that these pearls were from the pre- to early Columbian era.
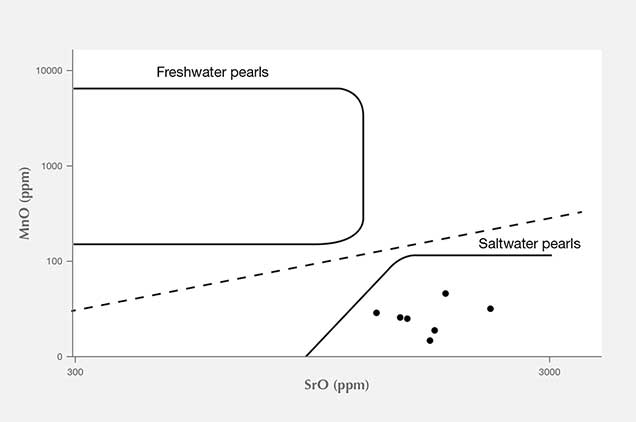
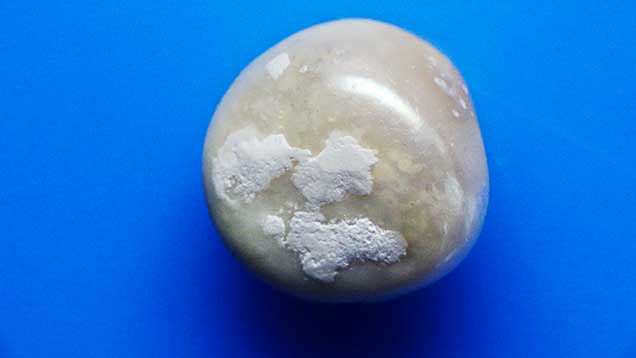

DISCUSSION
The radiocarbon dating of pearls that formed between the mid-nineteenth century and very recently has been reported previously. The results were found to be useful in providing additional valuable information on the age, provenance, and/or identification of those pearls (Krzemnicki and Hajdas, 2013). Testing in this study at different AMS facilities confirmed that the samples were from the pre- to early Columbian era. Local marine reservoir corrections were applied to the results obtained from each institution with the assumption that the pearls originated from the waters of the Caribbean Sea, as claimed by the supplier. Results from the different laboratories determined the approximate ages within fairly consistent time frames.
It is interesting to note that radiocarbon dating results indicate that the pearls appear to be of various ages, with some possibly preceding 1541. That was the year a hurricane and a possible tsunami destroyed the town of Nueva Cádiz on Cubagua (O’Loughlin and Lander, 2003), suggesting that the pearls may have been hidden in Cubagua, if the client’s claims are true, after the hurricane struck. Finally, it is worth mentioning that the variation in the results obtained from the different laboratories is common in radiocarbon dating experiments, as was shown in the famous case involving the Shroud of Turin (Damon et al., 1989). The slight differences between AMS laboratories may arise at several points, likely stemming from differences in cleaning protocols.
To summarize, both gemological and advanced chemical analyses confirmed that these were natural saltwater pearls, probably from a Pinctada species mollusk—e.g., Pinctada imbricata, which were harvested en masse during the Spanish colonization—if the reported geographic origin of the pearls is accurate.
CONCLUSION
This joint study has demonstrated that radiocarbon dating may play a pivotal role in determining the age of pearls—and possibly supporting the provenance determination—and distinguishing between natural and cultured pearls. This technique requires a small amount of sample powder (as low as 5 mg) to be extracted and analyzed and may have limitations with pearls of unknown geographic origin. Freshwater pearls may pose additional challenges due to the underlying geology of the watershed (limestone, for example). Pearls formed after 1955 also have atomic bomb–derived radiocarbon levels that make obtaining accurate results even more challenging.
The pearls and shell powders from which the samples in this study were taken were reportedly found off the Caribbean islands and were believed to be from the pre- to early Columbian era. Routine gemological studies confirmed they were natural saltwater pearls that probably formed in a Pinctada mollusk genus. Radiocarbon dating experiments conducted by three different institutions were in agreement with the claimed ages of the samples (within acceptable tolerances) and indicated that the pearls originated from a period when European colonists had just discovered the Americas (the late fifteenth to seventeenth centuries). The fact that these were not shipped back to Europe suggests that they belonged to Native Americans or colonists living in the region. Given their age, it is remarkable how well preserved these pearls are after spending centuries in unknown conditions.